Our interdisciplinary approach to catalysis
The Scott group at UCSB designs catalysts for large-scale chemical transformations related to energy, the environment, and sustainable chemical manufacturing. We aim to understand the interactions and transformations of molecules in solution and at gas-solid interfaces by studying the nature and behavior of active sites. We apply techniques from molecular chemistry, surface science, spectroscopy, kinetics, mechanisms and modeling. Our group includes both chemical engineering and chemistry students, working to solve important current problems at the interface of chemistry and reaction engineering.
Read more about our research projects below!
Upcycling Plastic Waste to Value-Added Chemicals
Every year, millions of tons of plastics are used and then quickly discarded as waste, creating a growing environmental burden due to their long lifetimes. Existing mechanical recycling methods generate lower value materials are economically challenging. With the Institute for Cooperative Upcycling of Polymers (iCOUP) and Prof. Mahdi Abu-Omar (UCSB), we are developing new chemical recycling methods could motivate better plastic recovery and reduce inputs to the environment.
Tandem hydrogenolysis/dehydroaromatization to alkyl aromatics
We discovered that waste polyethylene (PE) can be converted to molecular alkyl aromatics at moderate temperatures by coupling endothermic dehydroaromatization with exothermic hydrogenolysis:
Field desorption mass spectrometry
Designing better depolymerization catalysts
More active and selective catalysts were developed by making metal and acid sites act synergistically in a bifunctional catalyst. Halogenated aluminas are much more acidic than native alumina, and promote the catalytic cracking of olefins formed by alkane dehydrogenation on Pt sites.
Effect of catalyst Brønsted acidity on aromatic yield
The bifunctional reaction mechanism
Ethenolysis of waste polyethylene to propylene
Depolymerizing PE can generate propylene which is the monomer for polypropylene (PP, a commodity plastic typically made from fossil carbon). The catalytic transformation of PE is achieved by repeated cycles of transfer dehydrogenation (to generate olefins), ethenolysis (olefin metathesis, to cleave the polymer chains), and olefin isomerization (to convert terminal to internal olefins).
CH3ReO3/Cl-Al2O3 is an effective catalyst for both ethenolysis and isomerization. Propylene from monounsaturated PE is formed with 95% selectivity at 50% PE conversion. High selectivity to propylene shows that depolymerization occurs via "chain subtraction", starting from the chain ends and proceeding towards the center.
A preliminary life cycle assessment (LCA) comparing this method with conventional propylene manufacturing predicted a potential reduction in greenhouse gas emissions equivalent to removing 3 million gas-powered cars from the roads, if even 20% of the world's waste PE were converted to propylene in this way.
Research Sponsors:
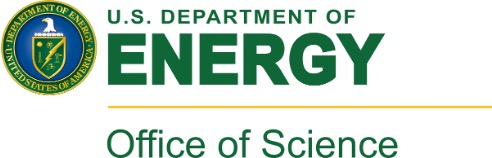
Probing Solid Catalysts using Advanced Solid-State NMR Methods
P-zeolite catalyst for biomass conversion
P-modified zeolites show high selectivity in acid-catalyzed reactions for biomass upgrading to renewable chemicals, such as conversion of furanics to butadiene or p-xylene. With he Catalysis Center for Energy Innovation (CCEI) and Prof. Songi Han (UCSB), we studied the nature of the P-sites and their dynamic behavior under reaction conditions, using solid-state NMR methods with advanced pulse sequences to distinguish sites with similar chemical shifts, and the enhanced sensitivity of Dynamic Nuclear Polarization (DNP) to reveal spacial correlations.
F-alumina for polymer upcycling
Solid acid catalysts have also been known to cleave C-C bonds for polymer upcycling. We've shown that the distribution of surface hydroxyls on the catalyst is modified with the addition of fluorine.
Research sponsors:

Designing High Performance Catalysts for Olefin Metathesis
Olefin metathesis is the redistribution of hydrocarbon chain lengths by rearranging the substituents on C=C bonds, for example:
It is widely used in manufacturing commodity chemicals, surfactants, polymers, and many specialty chemicals.
Atomically-dispersed catalysts (typically, based on Mo, W, or Re) on oxide supports (typically, silica or alumina) are highly active heterogeneous metathesis catalysts, in which the active site is a metal alkylidene. We are investigating structure-activity relationships at the molecular level in order to design more efficient catalysts.
A significant primary kinetic isotope effect suggests that the perrhenate activation mechanism involves C-H activation:
The oxide support affects the formation and stability of the active sites via interactions with Lewis and Brønsted acid sites. For example, chlorination of alumina results in dramatic increase in both activity and stability for a catalyst based on grafted CH3ReO3:
Catalyst activity and stability are enhanced by adding more support (such as Cl-Al2O3 or γ-Al2O3) to the catalyst. More active sites are formed when mobile CH3ReO3 migrates onto the new support:
Read about our recent work in: J. Phys. Chem. C 2021, 125, 23115; J. Am. Chem. Soc. 2018, 129, 8912; ACS Catal. 2018, 8, 1728; ACS Catal. 2017, 7, 7442; J. Am. Chem. Soc. 2016, 129, 8912; J. Phys. Chem. C. 2011, 30, 133; Top. Catal. 2011, 30, 133; J. Am. Chem. Soc. 2007, 129, 8912; Organometallics, 2006, 25, 2157
Research sponsor: 
Catalyst Characterization by Operando X-ray Spectroscopies
Using operando spectroscopies, we observe catalytically active sites while a reaction is taking place. This strategy ensures that active sites are in catalytically relevant forms.
Oxide-supported Ga catalysts promote the direct dehydrogenation of propane. The active sites may be Ga(I) or Ga(III). We recorded the X-ray absorption spectrum (XANES and EXAFS) in the presence of propane, propene, and H2 at the Stanford Synchrotron Lightsource.
Read about our recent work in: J. Phys. Chem C. 2021, 125, 27901; J. Phys Chem. C 2021, 125, 23115; ACS Catal. 2018, 8, 7566; J. Phys. Chem. C. 2015, 119, 26611; J. Energy Chem. 2013, 22, 1.
SSRL Beamline 2-2 is equipped with a goniometer to allow quick EXAFS scanning to study reactions.
Stanford Synchrotron Lightsource (SSRL)
In situ X-ray cell for recording spectra of air-sensitive samples at elevated temperatures.
Research sponsor: 
Dynamic Catalytic Condensers for Programmable Catalysis
Catalytic condenser with a thin graphene electrode to distribute electrical charge to catalytically active metal nanoparticles
AFM image of 2-5 nm platinum nanoparticles on a catalytic condenser
Using novel catalytic condensers, we store electrical charge in catalytic active sites to influence adsorbate binding energies and promote reaction rates
SSRL beam line 11-2 is equipped with a rotating state for in-situ XAS experiments at grazing incidence angles
ACS Catal. 2020, 10, 12666
Research sponsor:
Exploring Catalytic Mechanisms in Upgrading Biomass to Renewable Fuels and Chemicals
Selectively 13C-labeled model compounds such as 2-phenoxy-1-phenyethanol are synthesized to study the mechanisms of catalytic lignin conversion to aromatic monomers. Operando solid-state NMR studies of the reaction progress at elevated temperatures and pressures reveal the evolution of individual species in the reaction network.
Read about our recent work in: Chem. Sci. 2020 (in press); Green. Chem., 2020, 22, 550; J. Am. Chem. Soc. 2019, 141, 17370; ACS Catal., 2019, 9, 7204; ChemCatChem, 2019, 11, 190; J. Phys. Chem. C, 2018, 122, 8209; ACS Catal. 2017, 7, 3489; ACS Catal. 2016, 6, 8286; Catal. Sci. Technol. 2015, 5, 1540; ACS Catal. 2014, 4, 2165; Angew. Chem. Int. Ed. 2013, 52, 10349.
Research sponsor: 
Tuning Porous Catalysts for Selective Liquid-Phase Reactions
Solid catalysts for the efficient conversion of non-food biomass (lignocellulose) to platform chemicals should selectively adsorb reactants and promote desorption of products. By modulating the surface polarity of an ordered mesoporous silica (SBA-15) using various (organo)silica precursors, we have established that molecular adsorption can indeed be precisely tuned by a judicious combination of surface polarity and solvent. We are exploring the effect on catalytic activity and selectivity in the hydrogenation/hydrogenolysis of lignin model compounds.
Read about our recent work in: Chem. Sci., 2020, Advance Article
Research sponsor: 
Designing Catalysts for Continuous Flow Pharmaceutical Production
Pharmaceutical manufacturing is mostly conducted in batches, generating large amounts of catalyst and solvent waste, and requiring extensive product purification to remove catalyst residues. We are developing solid acid catalysts for more efficient pharmaceutical processes using continuous flow reactions to enhance safety, efficiency, and productivity.
In collaboration with Pfizer, we have found that robust materials such as H-Beta zeolite (HBEA) and amorphous silica-alumina (ASA) are effective solid catalysts for important pharma reactions such as:
- N-Boc deprotection of amines
- direct amidation of esters
- synthesis of N-substituted azacycles